Evolution of Flight in Bats
Winston C. Lancaster, Ph.D.
Department of Biological Sciences, California State University, Sacramento
Few activities of animals have attracted people’s attention and imagination quite as much as flight. Certainly no one who has spent a day struggling up a mountain ridge and paused to see an eagle soar across the same distance in less than a minute has failed to be struck with awe and envy. Whether we admire the speed, the freedom, or the appearance of ease, few could deny the attraction and fascination of animals that defy gravity to fly through the air.


As scientists, biologists strive for objectivity, but most of us were attracted to the field by the awe which we experienced the natural world as children, and carried this into our lives as adults. The objective requirement of science requires that we propose hypotheses and ask testable questions. If we focus our scientist's eye onto the question of flight, we might ask, what exactly defines flight? What animals fly? How many times has flight evolved? What were the intermediate stages of structure and function between a terrestrial ancestor and its freely flying descendant?
Powered Flight
Powered flight is characterized by an animal’s ability to alight from some surface and gain and sustain altitude by its own physical effort. This is distinct from gliding in which an animal might launch itself from a high perch and travel through the air at a low angle of descent to a controlled landing, but never gain height through its own effort. Gliding has arisen independently vertebrate animals in the bony fish, in amphibians, in reptiles and in mammals, and presumably in the earliest birds, though there we face ambiguities between where the birds start and the reptiles leave off. Among mammals, gliding has arisen in at least five separate groups. Powered flight, on the other hand, is much rarer. Sustainable, powered flight has evolved in the vertebrates unquestionably only twice, in birds and bats (opinion varies on whether pterosaurs were active fliers or spectacular precursors of today’s hang gliders).
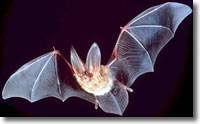
To further reduce the question, we might begin to ponder the stages of development between a terrestrial, quadrupedal animal and a flying bird or bat. One fundamental axiom of evolutionary biology is that every species that has ever existed must have been fully functional, well adapted and competitive for resources in its environment. If we think of species that were transitional forms between the highly adapted forms we know today (be they dolphins or bats for example) and their ancestors which were terrestrial mammals, each of these species which from our perspective were transitional, were in their own day the height of evolutionary specialization. They had to compete for food and mates, and had to evade predators and avoid harsh weather. With these parameters in mind, lets consider the evolution of flight in bats, the only group of mammals to have evolved powered flight.
A Gliding Ancestor
For any terrestrial animal, it is considered that flight can have developed in one of two ways: by going from the “ground-up”, by running and launching into flight, or from the “top-down” by climbing to a height and launching into the air. Looking at bats today, with their small legs with wings attached to them, it is difficult to conceive of a functional predecessor that could have run on its hind limbs like a bird, but with functional wings attached to them like a bat. Most evolutionary biologists who have studied the question agree that bats probably arose from a quadrupedal, gliding ancestor that climbed into trees or other heights and launched them selves into the air.
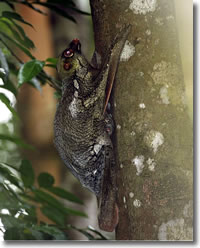
Gliding mammals generally have an expanded fold of skin from the trailing edge of the forelimb extending to the hind limb. Gliders with flight membranes like this are found in two families of rodents (like our flying squirrels), three different types of Australian marsupials and the amazing Colugo (or Flying Lemur, which neither is a lemur, nor does it truly fly). In the various forms, this glide membrane may stretch from the elbow to the knee, or from the wrist to the ankle. Some include webbing between the fingers. Some even have unique, extra bones extending form the wrist to allow for a wider wing, but also one that can change in shape to allow for steering. These gliding membranes are remarkably similar to the wings of bats, but are thicker and covered with fur. They have a narrow wing span, but the membranes are long from the head to the tail. This relationship of width to length, called aspect ratio, is a strong determinant of wing function, and gliders have a low aspect ratio.
Wings With Fingers
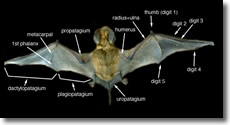
Bat’s wings are made of a supple, hairless, elastic skin stretching from the edge of the forelimb all the way to the tip of an elongated little finger. The wing attaches to the lateral side of the body, and lower limb down to the ankle. Some bats also have a membrane between the legs and connecting to the tail. This integration of the hind limb into the structure of the wing is unlike the wing of any bird, but is shared with gliders. The feature that is unique to bats is the extreme elongation of the fingers (“index” finger through little finger), with the membrane extending between each. All together, the bones of the hands and fingers are usually about as long as the forearm and so the skin between them (sometimes called the hand wing) effectively doubles the length of the wing over the part attached to the limbs and body. This results in a wing of much higher aspect ratio than the wing of a glider. The similarity between the wings of all living gliders and those of bats, all having evolved independently adds further circumstantial evidence to the concept that bat’s wings evolved from a gliding mammal ancestor. Despite the similarities, they are very different wings; they function differently, have certain advantages and different limitations.
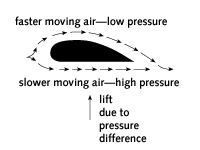
A glider does not flap the flight membrane. The wing is stretched tightly between extended fore and hind limbs. Lift is created by differences in air pressure above and below the wings as air moves across the wing. The animal launches himself into the air often with the body held at a high angle with respect to the direction of travel (called the angle of attack). This works well for their low aspect ratio wing. It generates excellent lift and has less tendency to stall (experience a sudden loss of lift) at the high angle of attack than a wing that is high in aspect ratio. However, this wing does not generate any lift by flapping; the narrow shape of the glider’s wing is not effective for flapping. To make a wing that can generate lift by flapping, the shape must change.
Aspect Ration Changes
With its long, thin, flexible fingers, the bat’s wing has a much wider span than that of a glider and is shorter from front to back; it has a high aspect ratio. This wing has a greater danger of stalling if the angle of attack is too high, but it can produce thrust by flapping while maintaining lift. The narrow, pointed wing tip generates less drag in the air while flapping than would a long wing edge resulting in a wing that keeps the animal in the air and also propels it forward. This is true powered flight, but it takes us back to the great evolutionary question: How were intermediate forms able to function? How was the transition made from the narrow, static, low aspect ratio wing of gliders to the wide, flapping, high aspect ratio wing of bats?
Of course, we will never know exactly; the fossil record by its very nature is incomplete, and flying animals are notoriously delicate and seldom found as fossils. One hypothesis comes from an observation of that some gliders do move their wings while flying. They are known to alter positions of their limbs to steer in the air. Furthermore, as the fingers of the precursors to bats began to elongate, the aspect ratio of the wings would change as well. These extensions could have been used to steer, and possible flap in certain situations. For example, speed, lift and angle of attack are all interrelated. One could imagine a gliding, protobat approaching a landing as its airspeed decreased and flapping the wings to steer slightly or ease into a gentler landing. Such small changes could effect the success of a high-risk strategy such as launching one’s self into from a tall tree in hopes of a smooth landing.
Bat Speed
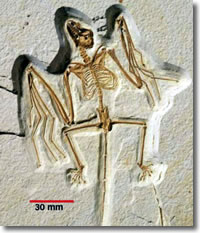
One problem with unraveling the mystery of the evolution of bats is the speed at which the transition occurred. The earliest known bat, Onychonycteris finneyi, was recently described from the early Eocene of Wyoming (about 52 million years old). While primitive by the standards of modern bats Onychonycteris had the wings of a fully functional, flying bat. Unlike the wings of modern bats, each of its digits had tiny claws, suggesting that it could climb in trees - perhaps hanging beneath small branches while searching for insects or moving to a good launching point. However, studies suggest that this bat was fully capable of flight. The lack of transitional fossils between the terrestrial predecessors of bats and the fully developed wing of even a primitive flying bat suggests that the evolution was very rapid in geologic time.
Enormous genetic changes had to occur to transform a small quadrupedal insect-eating shrew-like mammal to a bat capable of powered flight. Among these were the acquisition of the initial wing membrane between the body and the limbs, the elongation of the fingers and retention of the skin webbing between them, the loss of their claws (except for that on the thumb), and the change of the hind limbs from those used for walking to ones that could suspend the bat in the head-down, feet-up posture used by bats. The predecessors of bats had to alter their physiology to meet the great energetic demands of flight and at some point develop not only the means of using the faint echoes of their calls for navigation (echolocation, or biosonar), but the brain function to use these sounds to detect insects in free flight, calculate their speed and trajectory, and then use their amazing new wings to pursue and intercept their prey in free space, in the dark.
Bats have stimulated both scientific research since the 18th century and fascination of humans since we first looked up into the night sky. Scientific progress requires objectivity, but detached neutrality can never inspire the work and effort required to achieve scientific discovery. It is the inspiration that we feel at seeing animals perform feats that we cannot, it is the wonder of a child at the flight of a bee that plants the seed that can grow into the discoveries of science.
Sources
Bishop, K.L. 2008. The evolution of lfight in bats: narrowing the field of plausible hypotheses. The Quarterly Review of Biology 83: 153-169.
Giannini, N.P., A Goswami, and M. Sánchez-Villagra. 2006. Development of the integumentary structures in Rousettus amplexicaudatus during late embryonic and fetal stages. Journal of Mammalogy 87: 993-1001.
Simmons, N.B. 1995. Bat relationships and the origin of flight. Symposia of the Zoological Society of London 67: 27-43.
Simmons, N.B., K.L. Seymour, J. Habersetzer and G.F. Gunnell. 2008. Primitive early Eocene bat from Wyoming and the evolution of flight and echolocation. Nature 451: 818-822.